Quantum Computing, Sandwiches, and Superconductors | Chaos Lever
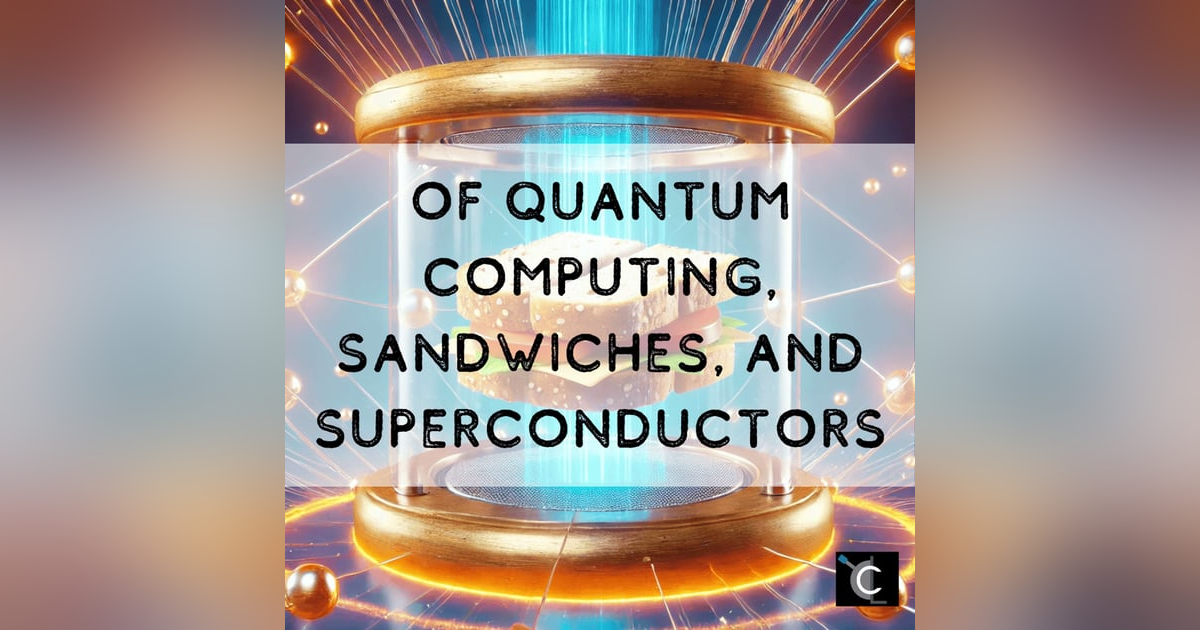
This week on Chaos Lever, we explore a heartwarming yet launch into an in-depth (and completely correct, don’t question us) discussion about quantum computing and the hardware solutions behind a qubit. 🧠⚛️
Google, IBM, Amazon, and even Microsoft have been making big moves in quantum tech, each promising advancements that may or may not totally destroy encryption as we know it. Superconducting qubits, quantum tunneling, and the mysterious Majorana zero modes—it’s all here, and it’s all *probably* real.
Stick around for deep dives into how different qubit architectures compare, what quantum error correction means for scalability, and why tech companies are obsessed with giving their chips weird animal names. If you make it to the end, congratulations—you've earned yourself a snack from the fridge, preferably one that doesn’t require quantum coherence to stay intact. 🍕
---
📌 **LINKS**
🔗 Superconducting Qubit Physics: https://web.physics.ucsb.edu/~martinisgroup/classnotes/finland/LesHouchesJunctionPhysics.pdf
🔗 Google's Willow chip: https://blog.google/technology/research/google-willow-quantum-chip/
🔗 Microsoft's Majorana chip: https://azure.microsoft.com/en-us/blog/quantum/2025/02/19/microsoft-unveils-majorana-1-the-worlds-first-quantum-processor-powered-by-topological-qubits/
🔗 Amazon's Ocelot chip: https://www.technologyreview.com/2025/02/27/1112560/amazon-quantum-computing-chip-makes-its-debut/
🔗 IBM's Heron chip: https://newsroom.ibm.com/2024-11-13-ibm-launches-its-most-advanced-quantum-computers,-fueling-new-scientific-value-and-progress-towards-quantum-advantage
🔗Topological state of matter paper: https://journals.aps.org/prb/pdf/10.1103/PhysRevB.107.245423
🌀 Thanks for listening! Follow Chaos Lever for more questionable but entertaining tech discussions. See you next week! 🚀
00:00 - - The LinkedIn sandwich dilemma
01:25 - - Fluffernutters and sandwich snobbery
03:15 - - The future of our artisanal sandwich empire
04:52 - - Quantum computing: It’s back, baby
05:12 - - What the hell is a qubit?
12:49 - - Superconducting qubits and their weird physics
19:22 - - IBM Heron vs. Google Willow
32:44 - - Amazon’s Ocelot chip: Do they even know what a cat is?
36:49 - - Microsoft and their theoretical qubit magic
39:05 - - Final thoughts & your well-earned snack
[00:00:00.06]
Ned: And then I got this message from somebody that I accepted a connection request from that said, I know I'm a stranger, but could you please help me out? I'm desperate. And I'm like, Well, maybe he has a real difficult technical problem that he's trying to work through. I'm like, Sure. Can you give him a little more context? And then his response was, Thank you for replying, I'm homeless, but mainly starving. I haven't eaten in eight days. Can you help me get a sandwich, please? I'm ashamed to ask. I don't know what to say about that.
[00:00:44.18]
Chris: That It's a tough one.
[00:00:47.08]
Ned: It's a tough one. I wanted to respond, did you try sudo make me a sandwich?
[00:00:59.00]
Ned: Hello, Alleged Human, and welcome to the Chaos Lover podcast. My name is Ned, and I'm definitely not a robot. I'm a real human person who does not beg you for sandwiches over a LinkedIn, mostly. So if you had a Fluffernutter, I wouldn't turn it down. With me is Chris, who's also here. Chris, why do you love Fluffernutters so much?
[00:01:25.03]
Chris: I'm going to answer your question with a question. Do you respect Do you accept that it's actually very hard to make a good fluffer nutter?
[00:01:39.10]
Ned: I do accept that. I accept that there's a challenge there. I'm going to say it mostly has to do with the ratios.
[00:01:47.06]
Chris: Correct. And what do most people do?
[00:01:50.16]
Ned: They put way too much marshmallow fluff on there.
[00:01:55.00]
Chris: Correct.
[00:01:55.23]
Ned: You think you want it all, but you do not. You do not. A third of the way through that sandwich, you're like, Ah, shit.
[00:02:04.02]
Chris: I didn't know that you could get diabetes in a 12-minute period.
[00:02:09.10]
Ned: Here we are.
[00:02:13.05]
Chris: It's the same problem with peanut butter and jelly sandwiches. I mean, I think it's actually worse with the Fluffernutter because the sugar content is so freaking high.
[00:02:21.11]
Ned: Yes.
[00:02:22.12]
Chris: But people that don't like Fluffernutters and people that don't like peanut butter and jelly, first of all, you're probably eating the wrong peanut butter.
[00:02:30.15]
Ned: Smooth. Immediately problem. Don't eat smooth. That was your first mistake. Correct. I'm so glad. We should open a sandwich shop. We sell two sandwiches, and there's only one way to make each.
[00:02:43.18]
Chris: And each one is 78 dollars.
[00:02:46.17]
Ned: You know, if we open that in Brooklyn, we would probably make a shit ton of money. Ironically. It's just the right level of... It's very twee. It's our way or the highway, so we refuse to customize, and it's very bespoke and expensive. Yes, that's everything we need. So I guess this will be our last episode of Chaos Lever, because we're going to go become Sandwich Lords of Brooklyn.
[00:03:15.15]
Chris: Congratulations. Sandwich Lords of Brooklyn does sound like an Apple Plus show.
[00:03:20.03]
Ned: That'll be what we do after we open the sandwich shop is get our biopic on Apple TV. But I guess before that, we should talk about the thing that I wrote 2,700 words about.
[00:03:35.22]
Chris: I admire your restraint, Ned, as usual.
[00:03:39.05]
Ned: Seriously, as we'll see as we bring this in for a landing, I could have just kept showing, but I looked at the page count and said, Nay, not today, sir. It looks like it's my turn to add to the ever-growing compendium of episodes we've done around quantum computing. This This particular one was largely spurred by the announcement of quantum chips from at least three major tech companies in the last few months. Once I looked back, it was four or five tech companies that were like, We have a quantum, too. With all the hubbub around AI, quantum stuff has taken a back seating the zeitgeist, but not here at Chaos Lever, where we find solace in quantum computing. Can you see what I-I'm disappointed in you, and you're only on word 30. I should add that I've never actually seen that James Bond movie. In fact, the last one I saw was Casino Royale, which was fine. It was fine. I'll always be more of a Roger Moore person because I'm old and I'm lame.
[00:04:52.23]
Chris: Accurate.
[00:04:54.01]
Ned: Okay, thank you. I appreciate the confirmation. Rather than trying to explain what quantum computing is and the algorithms behind it, I thought we could take a look at the hardware that drives this branch of computing. Starting with, the hell is a qubit anyway?
[00:05:12.15]
Chris: Isn't it that game where the guy jumps in a three-dimensional map?
[00:05:18.00]
Ned: It's two-dimensional, really. It just pretends to be three-dimensional, like all video games.
[00:05:24.06]
Chris: So does real life, man.
[00:05:29.23]
Ned: Just like its namesake, the bit, a quantum bit or a qubit, has two distinct states, on or off, one or zero. What makes it different from a digital bit is that a qubit can be in superposition i. E. In both states at the same time. Now, that doesn't mean that it's somewhere between. It's not a range. It is literally both one and zero at the same time. It can be entangled with other qubits to form a system of calculation. Criss, you spent a whole episode explaining this.
[00:06:04.06]
Chris: I was going to say, I don't want to go into it right now. We'll just go listen to the other one because we did talk about what the hell that actually meant. You won't get any more knowledge out of it, but it will spend another 35 minutes of your day.
[00:06:16.06]
Ned: Which you are obviously avoiding doing other things, so just continue down that path.
[00:06:22.01]
Chris: Eventually, the vacuuming will do itself.
[00:06:25.01]
Ned: Yeah. We did a whole episode trying to understand it. We wrap our brains around it, and instead, we ended up wrapping our brains around a gold brick with a twist of lemon. If you know what that's from, congratulations. Any material that can be used as a Qubit needs to exhibit three characteristics, two distinct states with no overlap, superposition, and entanglement. It has to have those three traits. But what makes it a good qubit? There are a list of desirable traits. The first one, and we have definitely brought this up before, is long coherence time. As long as a qubit can stay in superposition and entangled, it is coherent. Eventually, environmental factors will cause the Qubit to decoher and either return to a ground state or be disrupted by environmental noise. While it's coherent, you can do calculations with it. When it decoheres, you can't. That coherence time could be a few hundred microseconds up to several minutes, depending on the Qubit type. Longer is better. Right.
[00:07:43.17]
Chris: And compare that to standard classical computing, where a bit will stay in its state for as long as the electricity is on.
[00:07:52.05]
Ned: Yes. If it's not a static form of storage, in which case it'll stay bit until it rots out in however many hundred years. The next one is high scalability. This is how many qubits you can add to a system while maintaining low error rates and noise. It also requires that you successfully connect the qubits and facilitate communication between them. Oh, and you have to control this God forsaken mess and get useful data out of it. Scaling is really challenging. Basically, you have to solve You have to solve the coherence thing, but then you have to solve the scalability thing. Even if you get one good qubit, can you make a lattice of qubits? Can you make logical gates from them? Does that system scale? Generally, the answer so far has been no. The next one is high fault tolerance. Now, this is more a function of the system as a whole, but it's basically the ability to deal with individual qubit failures like decoherence. If you're running something in a fault-tolerant way, even if one qubit fails, you have a mirrored qubit that's somehow taking its place.
[00:09:09.23]
Chris: Or something.
[00:09:11.08]
Ned: Or something. Next up is initialization, because before you can do anything useful with a qubit or the system as a whole, it has to be placed in an excited state and primed for loading the computation that it's going to do. It's like setting all the transistors to the same state when you restart a computer. But it's a lot more difficult because these are qubits and they're finicky. Number 5 is universal quantum gates. The easiest way to think about this is think about classic logic gates that you would use in a processor to build circuits.
[00:09:53.23]
Chris: Like everyone does every day.
[00:09:56.07]
Ned: Yeah, I mean, I've got a breadboard right here, don't you? Yeah, but my lunch is on. We did a whole episode on this. It was called Classical Computing 101. God, this is very episode reference heavy. It's like a clip show. It's not a bottle episode. In Except every episode is a bottle episode. In that episode, we talked about the fundamental gates, the and, or, and not gates, and how you can build a half adder and then a full adder using these gates in a transister. That forms the basis for an arithmetic and logic unit in a CPU package. That's classical computing, but the same idea applies. So qubits need to be formed into quantum gates to handle quantum algorithms. A couple of examples are the Clifford plus T gateset and the Toffoli plus Hadamard gateset. Don't ask me to explain what those are because I tried to I read it and my eyes started crossing and I was like, This is not good. It also doesn't help that all these things are named after people instead of just obvious and or not. I don't like that. Probably the people who came up with it like it, though.
[00:11:17.01]
Chris: Yeah, I think they probably do.
[00:11:19.23]
Ned: Feeling very satisfied with themselves.
[00:11:23.11]
Chris: Oh, we're going to set up another me gate. Exactly.
[00:11:29.07]
Ned: The next one is efficient measurement. Quantum computing isn't very useful if you can't measure the results of an operation and do so in a reasonable amount of time, i. E. Before the system decoheres. The way in which results are measured is determined by the It's the Qubit type and the structure of the system architecture. It tends to involve a lot of microwaves for some reason, although sometimes photonic sensors. Then lastly, faithful transmission. This is the movement of information between qubits, and When that's required to perform successful computation, that transmission needs to be error-free, preserve the quantum state, and avoid duplication. That's a list of desirable traits. Some of them are in tension with each other. Depending on the material and the approach used for constructing cubits, some of the traits will be favored more than others. For example, superconducting cubits have a very high scalability factor, but extremely only short coherence times. Trapped ion cubits have long coherence times into the minutes range, which is pretty huge for quantum computing, but they have severe scaling challenges due to complex trapping and laser systems that are used to reset and read the contents of the system.
[00:12:49.10]
Ned: They use freaking laser beams.
[00:12:52.22]
Chris: Freaking laser. You're telling me we have a system where we can get an answer, but we can't read the answer?
[00:12:57.13]
Ned: We have a system that is not scalable. That's the biggest problem because it's very difficult to trap the ions in the Qubit using the lasers, and then to read that information out. You can do it with a couple, but as you try to scale it up, then getting the laser to reach each individual qubit becomes much more difficult. There are seven main approaches for building qubits, some more theoretical than others. We're not going to get to all seven. Otherwise, this would have been a six-hour podcast. I know everybody wants that, but not today. The most popular one, the one we're going to talk about the most, is the superconduct cubits. But there's also quantum dots, trapped ions that I just mentioned, photonic cubits that use light and photons, in the name, defect-based cubits, topological cubits, and nuclear magnetic resonance cubits, or NMRs. We're going to talk about superconducting and topological cubits today. I thought about going through each of these approaches and listing out their advantages and disadvantages, but A, that's super dry and not exactly conducive to listening unless your goal is to fall asleep. Instead, I thought, let's just look at some real chips that are out there and the Qubit approach they're actually using and how well they're doing.
[00:14:30.14]
Ned: Perfect. Okay.
[00:14:33.05]
Chris: Toastitos. Wait.
[00:14:38.03]
Ned: I was going to make a talkies joke, but it's too late. Chris, can you think of the earliest quantum computing chip you ever heard of?
[00:14:55.01]
Chris: That wasn't a question. That was not even a complete sentence.
[00:14:58.09]
Ned: What is the earliest quantum computing chip you ever heard?
[00:15:02.14]
Chris: Ibm.
[00:15:04.11]
Ned: Interesting. Okay. We are going to talk about IBM, but first, I want to mention D-wave. That's because I remember a bit of a hubbub, a little controversy over D-wave's one and two chips, and whether they actually are real cubits. The reason behind that is that D-wave's chips don't implement universal quantum gates as mentioned earlier. The chips instead use what's called quantum annealing to solve complex optimization problems. Think of the traveling salesman problem. If you visit all the different cities in the most efficient order, the more cities you add, the more difficult the optimization is. Bin packing is another one. These are problems that may not have a single optimal solution, and the calculation of solutions is very expensive for classical computation. One possible solution for it is to use what's called magnetic annealing to find a solution using the intrinsic properties of magnets to find their own optimal minimized state. I barely understand any of this, but I'll try to explain.
[00:16:20.20]
Chris: You're doing good so far.
[00:16:22.11]
Ned: Thanks. You have a bunch of magnets on a grid, and they're placed in an initial state. The magnets are are allowed to flip their orientation to try to find an arrangement that minimizes the amount of overall energy stored in the field. Two magnets with the same polarity point at each other don't want to be doing that, so one of them will try to flip. Essentially, you're using physics to model a mathematical problem and then letting the model run. You can do this. But there are some problems. Classical annealing requires that the magnets slowly be cooled to temperatures near absolute zero, which continuously forces them to try to find a new optimal arrangement. But the arrangement can get stuck in a suboptimal position because there's not enough energy left in the system to flip to the more optimal one. What D-wave built was a chip that has a bunch of magnets on a grid, and those magnets are entangled, which allows quantum energy tunneling to go between the different magnetic dots at lower energy states. Even if you hit that suboptimal arrangement, the quantum tunneling lets it pass energy through the system to find an even more optimal result.
[00:17:48.00]
Ned: You just have to trust me that this works.
[00:17:51.12]
Chris: I'm smiling, nodding, and backing away as we speak.
[00:17:54.14]
Ned: That's the one. D-wave calls these magnetic cubits, but I don't know. It seems suspicious. I guess they're cubits, but really all they do is solve an optimization problem. They're not a general purpose quantum computing solution. If you're trying to solve optimization problems, D-wave, good fit. If you're trying to do anything else, maybe not such a good fit. Like I said, a little bit of controversy there, but they seem to be a successful company, so I guess somebody's buying their chips and using Now, let's move on to a processor that we've actually talked about before in one of the lightning rounds, the IBM Heron. This was announced in November of 2024. Let's start with the type of Qubit we're talking about here. The Heron is a superconducting qubit architecture with 156 qubits on the die. Ibm claims that they have virtually eliminated cross-talk in the qubits, which refers to the interference and environmental noise that can lead to errors and impact accuracy in the computations. That was one of the desirable traits is to remove that cross-talk between the qubits. How does a superconducting qubit work? Well, I guess we first need to talk about what a superconductor is.
[00:19:22.02]
Ned: Chris, do you know what a superconductor is?
[00:19:26.03]
Chris: It's the guy that drives the train, but he's really cool.
[00:19:30.04]
Ned: But he wears a cape.
[00:19:31.21]
Chris: I said cool.
[00:19:34.12]
Ned: Okay, fine. That is one type of superconductor, but we're going to talk about a slightly different one from materials science. If you think about a superconductor material, you probably think of something that's cryogenically cold, down to near absolute zero. There's billowing clouds of liquid helium surrounding it, those kinds things. That's true. But what a superconductor is, is basically the lack of resistance in a material when you apply a current to it. Normally, when you apply an electrical current to a material, it encounters some level of resistance from that material. Insulators have a very high resistance. Conductors have a lower resistance. Think of a copper wire. It's happy to transmit your electrons, but it still has some level of resistance. That resistance is measured in ohms. Essentially, it's the conversion of electric current into heat. As the electrons try to move through the metal, that causes some amount of the electrons to scatter through the pattern of the material. That scattering leads to the material vibrating, which is what produces heat. This is why resistors get warm when a current is passing through them. They're converting the electric energy into heat energy. The movement of electrons also creates a magnetic field because the two are closely related.
[00:21:13.15]
Chris: This is also why you need a heat sink on your CPU?
[00:21:17.06]
Ned: Exactly. Otherwise, it'll melt. It's the electrical resistance. Well, it's actually also just the gates changing, regardless. In a superconductor, the resistance drops to zero, and the current can travel through the material unimpeded. No heat is produced, no electrons are lost, and no magnetic field is produced either. It's interesting. This is some very weird stuff that's happening at the quantum level that enables superconduct. That's fun to say. In particular, the electrons that are traveling through as the current form pairs. They're called fosons. The bonds are called foson bonds, and the pairs are called Cooper pairs. They travel like holding hands through the material, which is why they don't scatter, because they're holding on to each other as they travel through the material. I swear this is all true, even though it sounds totally ridiculous and made up. It is a well-known phenomenon that as materials get When it gets colder, their electrical resistance drops, but it turns out that some materials resistance will just drop to zero when cooled below a critical temperature. Now, there are no room temperature superconductors, despite what you may have heard on TikTok, the highest critical temperatures of superconductors at the moment are in the 92 Kelvin or negative 181 centigrade range.
[00:23:00.16]
Ned: That's real cold.
[00:23:02.10]
Chris: I think that's what it was yesterday. Plus, it was windy.
[00:23:05.01]
Ned: It turns out this is just slightly above the temperature of liquid helium, which is why you can use liquid helium to cool these types of superconductors down to where they become superconductors, which is convenient. That's why we found materials that are in that range because it's relatively easy to make liquid helium.
[00:23:29.23]
Chris: Because if all you have is liquid helium, then everything becomes a superconductor. I think that's how that expression goes.
[00:23:39.12]
Ned: Yeah, that's it. That's the one. Get it printed on your tombstone. Now that we know a little bit about what superconductors are, why would they make good qubits? Listen, I'm not a physicist, so if you happen to be listening and you are a physicist, you probably turned this off by now, but please be gentle if you're going to continue listening. A qubit needs two discrete energy states, the one and the zero. It cannot have anything in between. You can get this behavior through a Joseph Sun Junction, which is hard to say.
[00:24:21.13]
Chris: That was not even hard to say.
[00:24:23.02]
Ned: It is for me. I don't know why. Joseph Sun Junction. The first time I've had to say it out loud. I've been typing it. This is created by taking two superconductors and separating them by a very thin insulator. Those Cooper-electron pairs can quantum tunnel across the insulator between the two superconductors, which forms a DC current. If the current exceeds a certain critical level, it begins to oscillate as an AC current. The transition in inductance of the junction is nonlinear, meaning it jumps from one discrete level to another. According to a paper from UCSB, The change of the qubit state from one photon in energy can modify the junction inductance by order unity, which is really what the paper says. I should note the exclamation mark on the end. I didn't add this. The nerves were really pumped about this. What What I think this means is photons have very little energy as an individual photon, but just one photon in energy change will modify the junction from one state to another. There really is no in between. It's either in one state or the other, and that's it. That's about as far as I can take it, and I'm sure I don't really understand it.
[00:25:55.19]
Ned: The resulting structure is a nonlinear resonator. It's not linear because it doesn't have a continuing range of values, one or zero. That can be achieved through three approaches. There's flux, charge, and transom. Each of these approaches leverages a different part of the equations that govern this weird circuit, this junction. And there are symbols involved that I don't even know the name of, Chris. I'm looking at him like, That's not even a Greek letter anymore. I That's an emoji of a crying man. It's me. I don't know what they were meant to represent, but according to the paper, out of all of them, the transom or phase cubit is the most commonly used because it has the highest coherence times. Now we know that superconducting materials are good for making qubits, and specifically this transom type of cubit is used most commonly because it has the highest coherence times out of all the different superconducting cubit types. Cool.
[00:27:07.03]
Chris: Cool.
[00:27:08.02]
Ned: All right. Now, how do you control this whole thing? The control element for superconducting cubits is microwave pulses. These are what set the state of the qubit for initialization. A resonator is coupled to the qubit, and its frequency shifts slightly due to what they call dispersive interaction, which, as far as I can tell, think of if you have two tuning forks and you bang one, so it starts resonating and you put the other tuning fork near it, that other tuning fork will start resonating in time to the one that it is close to. That's what the resonator is doing inside of this detection mechanism for the Qubit. It picks up on the resonance without actually disturbing the state of the Qubit. Then the resonator is what is actually probed by the microwave signal to measure what state the Qubit is in. Cool. In terms of pros and cons, the superconducting Qubit is the most common in the industry, more common than any of the other six options. The use of phase transitions allows for faster gate operations, and the fabrication of superconducting qubits can use existing manufacturing techniques for regular processors. The lithography that they use to make those processors, you can use the same thing to make these superconducting qubits.
[00:28:39.21]
Ned: You can create strong connectivity between qubits, which makes it easier to form logic gates. And the control and readout methods are well-developed and understood. We know how to make microwaves real good. And microwave burritos. Not just to make popcorns. Now I'm just hungry. Still, just because these techniques are understood doesn't mean they're error-free. Decoherence still happens on the microsecond time scale, which is really fast. There are very high level error rates, and there's issues with cross-talk, especially as the number of qubits in a system increases. Plus, the whole system has to be kept at pretty extreme temperatures. We're talking 10 to 20 millikelvin above absolute zero. That's what they keep the IBM Heron at. The IBM Heron is delivered in the IBM, I think it's Quantum System 2 is the name of the system, it is gigantic, and it includes all the cooling things that are meant to keep these chips at these ridiculously low temperatures. If you have a few hundred million dollars, you could buy one for yourself.
[00:29:59.02]
Chris: I think it would look nice in the living room.
[00:30:02.03]
Ned: The system actually do look pretty cool. I'm not going to lie. I guess if you're going to put that much money into it, you might as well make the exterior look half decent. All right. The next chip is called the Google Willow. This section is going to be shorter because the Willow chip was announced in December of 2024, not long after the Heron announcement. Just like the Heron, the Willow chip is also using superconducting qubits. This one has about 105 qubits compared to, I think, 156 for the heron. We don't have to go through what superconducting cubits are, again. What makes this chip better or different? Well, Google made some bold claims about the performance of Willow and what it means for the future of quantum computing. The first big claim is that it has a massive improvement in error correction, one that actually improves as they scale up the number of cubits. You think, usually, add more cubits, more errors, right? But the way that they have set this up with quantum error correction, the Willow Chip actually improves based off of scale, and they saw the number of errors reduce as they created larger grids of cubits.
[00:31:15.07]
Ned: If all of that is actually true and passes muster, it means they can build bigger systems with more cubits and stay below the error threshold, meaning they can run more complicated operations reliably. Speaking of that operation, their other big claim was how fast the chip was able to complete the random circuit sampling benchmark. This is the benchmark that Google uses for all of their quantum chips, and it says it should be the industry standard. Now, I don't want to go off on too much of a tangent because time, but IBM claims that the benchmark should be CLOps or circuit layer operations per second, which is different. How do these two approaches compare? I don't know. But that's probably going to be a whole other episode at some point. Anyway, Google says there will- The main takeaway is supposed to be that Google wants to use the benchmark that makes their chip look best. That's the one. And You wouldn't be surprised to discover- Not that a manufacturer would ever do that. No. You wouldn't be surprised to discover that CL Ops favors the heron.
[00:32:24.21]
Chris: Shocking. I am shocked.
[00:32:26.23]
Ned: Anyway, Google says that their Willow Chip can solve the benchmark in under five minutes, and that it would take a classic supercomputer 10 septillion years to do the same, which is far longer than the age of the universe.
[00:32:44.15]
Chris: Pretty sure that's also a fake number.
[00:32:48.03]
Ned: Probably. I think it was like a 10 to the 28th or something ridiculous. All right, sure. Next up, we have a chip that you can't buy. The Willow is a real thing that they're producing. Amazon announced the ocelot in February of 2025, and this chip is a prototype. There was a lot of faffing about in the PR, but as far as I can tell, it only has five functional Q-bits on it, and then five more cat Q-bits that are part of the error correction approach. Amazon's claim is that they have developed a new and novel approach to quantum error correction by rethinking the entire design of the chip. The ocelot uses traditional manufacturing techniques, but the devil's in the details, and those details are in a nature paper that I will link in the show notes because we ain't got time. Just like the Just like the heron and the willow, this is also a superconducting qubit architecture, which begs the question, there are like six other qubit types. What about those? Aha. That leads us to the last chip for this roundup. The Microsoft Maiorana or Maiorana, I've heard it pronounced both ways, from different Microsoft people, so it's like Azure all over again.
[00:34:10.20]
Ned: What a freaking nightmare. This chip is not using superconducting qubits like the previous entries. I know. If I may quote the Microsoft blog post from February 2025, The world's first topo conductor. This This revolutionary class of materials enables us to create topological superconductivity, a new state of matter that previously existed only in theory. That is correct, everybody. Microsoft has created a new state of matter called topological phases.
[00:34:49.22]
Chris: Or so they say.
[00:34:52.05]
Ned: The reason the chip is named Magerana is because that was the Italian physicist who first came up with the idea of topological phase matter. But like the quote says, that it was largely theoretical and hadn't really been created in the lab consistently. Microsoft claims that they have done this with the Majorana chip, and they have used this new state of matter to build Majorana zero modes that serve as the Qubits. Now, I'm hoping to have someone from Microsoft come on the show to explain what any of that means, since there's a lot of jargon in the archive paper that I tried to read from the blog post link. Here's a sample. In the case of quasi one dimensional superconducting wires without any symmetries enforced, there are only two phases, one trivial and one topological. The latter supports Magerano zero modes localized at the ends of the nanowire. If you had to write Techno Babel for a Star Trek episode, episode, you could not do better.
[00:36:03.19]
Chris: I might be mildly concussed.
[00:36:06.13]
Ned: And yet... Yeah. One dimensional, quasi one dimensional superconducting wires. I think we exist in 3D. At least last time I checked, and the only time I've ever encountered one dimensional stuff was in that book Flatland, and also in my eighth grade geometry class, where, again, it was purely a theory. Anyway, according to Microsoft, MZMs are new fundamental building blocks for qubits, and they store parity based on the number of electrons in the wire. The unpaired electrons are shared between two pairs of these MZM wires, making them invisible to the external environment and thus not subject to interference, which prevents some of the error creep that happens with traditional qubits. Microsoft uses microwaves to measure the parity of the MZM wire, and that is how they get the readout of the state of the Qubit. They claim that this vastly simplifies the necessary quantum error correction for their chip, and the measurement, along with the architecture, is scalable. In fact, the post makes frequent mention of a one million qubit system. Chris, do you think we're anywhere near that?
[00:37:26.11]
Chris: Maybe next week.
[00:37:28.19]
Ned: You They are adorable. The Majorana chip has eight qubits on it. Eight. Which, if I'm doing my math correctly, is somewhat less than a million.
[00:37:42.11]
Chris: What if you round up? I suppose.
[00:37:46.23]
Ned: Still, the Intel 4004 was released in 1971, and it had 2,300 transistors on it. If you fast forward to last year, the AMD Thread Ripper has something like 30 billion transistors on it. So like, Mohr's Law is a hell of a drug. All right. So final thoughts. Big round up here. I got through two of the seven qubit types. You had to know this wasn't all going to fit in one episode. I was pretty clear about that up front. Regardless of which architecture you choose, though, it seems like the biggest challenge at the moment is dealing with quantum error correction and scalability. Capability. If we want quantum computing to actually be useful, and by useful, I mean destroying all conventional encryption methods, we need to find a way to scale up systems while lowering the error rate. Will it be Google with their a silo chip, which is like an actual chip, or the more theoretical Magerana or ocelot. I don't know, but I suspect it will be a combination of approaches that eventually crack the quantum conundrum. Happily, they will still keep naming things in very dumb ways. Hey, thanks for listening or something.
[00:39:05.15]
Ned: I guess you found it worthwhile enough if you made it all the way to the end. So congratulations to you, friend. You accomplished something today. Now you can go sit on the couch, quantum tunnel into your refrigerator, and get yourself a hot dog. You've earned it. You can find more about the show by visiting our LinkedIn page, just search Chaos Lever, or go to our website, chaoslever. Com, where you'll find show notes, blog posts, and general Tom Foulery. We'll be back next week to see what fresh hell is upon from us. Ta-ta for now.
[00:39:43.01]
Chris: I looked up this Amazon chip real quick because I was curious. Apparently, the whole cat state is two different types of Qubits that both try to store the same information. It's like a team. My only question about this is, does Amazon actually know what a cat is?