Intro To Quantum 101, part 1: Light is Crazy
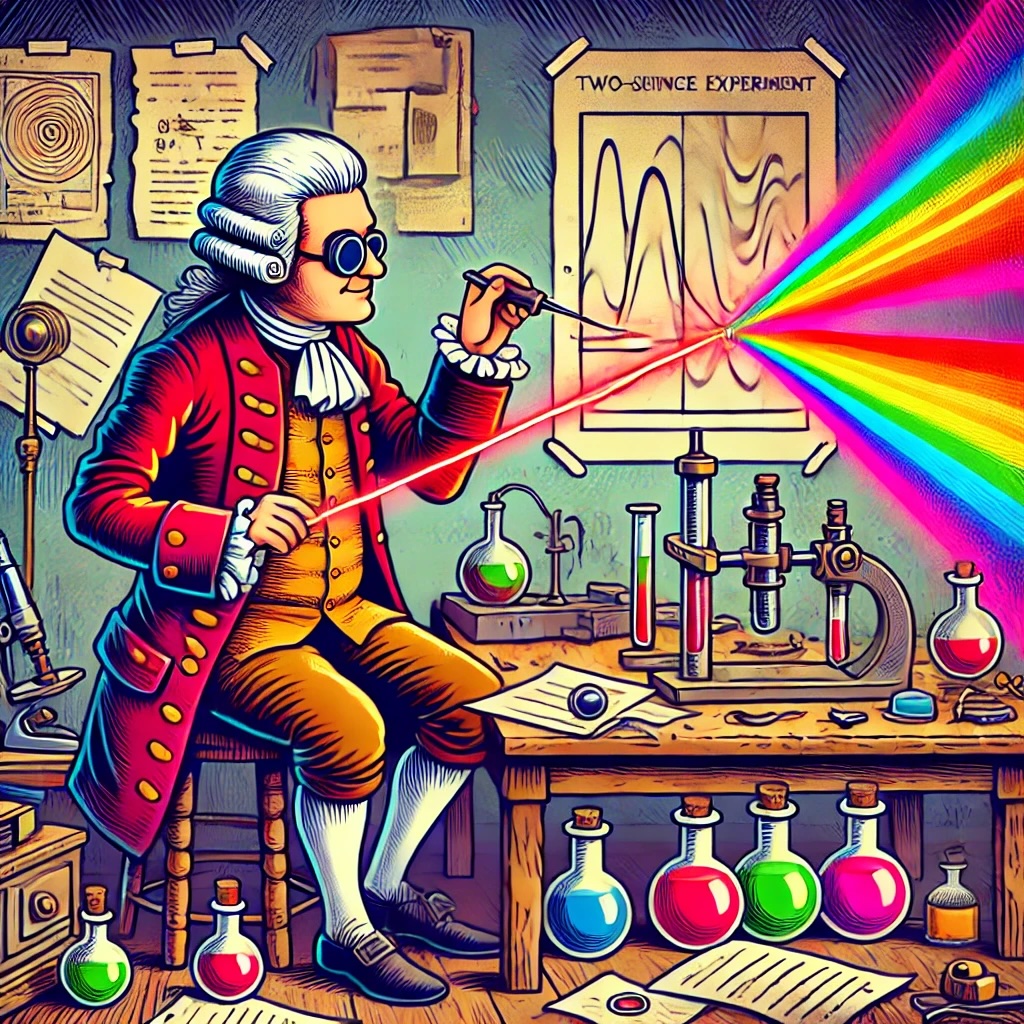
2025 Will Be The United Nations Year Of Quantum Science and Technology
So that’s pretty cool. They decided on 2025 because 2025 marks the officially unofficial 100th year since quantum mechanics became a real, formalized, and accepted… thing. And we should talk about it here- a blog that is ostensibly about computers- because without quantum mechanics, we wouldn’t have quantum computers. And quantum computers have two main characteristics: 1) they seem cool, and 2) the majority of people have no idea why or how they work. One major reason people don’t understand quantum computers, is that they don’t understand quantum mechanics.
Is that a stretch? Maybe. But we're going with it.
Quantum mechanics flies in the face of everything that seems logical about the world. Here’s an example quote describing the critical difference and weirdness about quantum computers: “The principle of superposition, fundamental to quantum mechanics, is what gives quantum computers their power. In a quantum computer, performing an operation on a qubit in superposition is equivalent to performing the operation on all possible values simultaneously.”
Got all that? Yeah. Me too.
But for everybody else who might need a little help understanding what is going on, how it’s possible, and how we figured all this out, I thought I would take a little gambol through space and time- but not spacetime, that's completely out of scope- and try to shed just a little light on how we got… wherever it is we are with quantum mechanics right now. And to do that we need to do a little history, but I swear to you that there’s no math. Well- there is math, but I’m not a mathemologist, so I’m gonna tell you what the math proves, without delving into an formulas. But trust me, they exist, they are real, and as many important people have said… they’re spectacular.
PART 1: Light History: Wave, or Particle … Orrrrrrrrrrrr?
One of the first major efforts to understand the world around us was trying to understand light. And it goes back a looooong time, because you don’t have to be a scientist to know that light is weird. Some light is harmless - like a 60watt bulb. Some light is so intense it can burn your skin. And light doesn’t even have to be generated by electricity or fire - go get a framing nail and a hard piece of wood and hit the nail hard a few times. Eventually it will start to glow. What on earth is THAT about?
Even the ancients knew this one - there’s a correlation between light and heat. Get something hot enough, and it glows. Get it hotter, and it glows a different color. So heat makes light? Or, think about the many different ways that light can be manipulated. It bends through water, it's split into many colors when it hits certain kinds of glass. It has all kinds of odd characteristics that are easily reproducible, but very challenging to explain- especially if you're trying to explain everything all in one system that explains everything.
I’m going to skip roughly 2,000 years of kind of interesting philosophizing on the topic and fast forward to the 1700’s, the lab of guy you might have heard of named Issac Newton. He posited the theory that light was a particle. It traveled in a straight line unless it interacted with matter, in which case it would bounce off. This was contrasting Christiaan Hugyens 1680’s idea that light was a series of waves that were emitted in all directions.
You can see why there was philosophical reasoning behind both positions, though, right? Wave theory was already understood to a certain extent, because even in the 1680s… we had ponds. Throw a rock in a still pond, and waves come out in regular pattern in all directions simultaneously, which, according to Hugyens, was just like light. Newton, by contrast, showed that he could explain reflection and refraction using particle based arguments. His argument held sway for a century, primarily because a) he was the hottest name in science and the most influential, and b) there wasn’t what we could consider ‘evidence’ that proved the case for either side.
PART 2: Light History: Enter Thomas Young
Fast forwarding again to 1801. A scientist named Thomas Young had an idea for a way that this could actually be tested. First, though, a thought experiment: lets say you have a flat piece of wood, say… 2 feet square. In the middle of the block, you cut two perfectly parallel slits, each one about a half an inch wide, and maybe a half an inch apart. Now hold that about a foot off the ground, and pour a bucket of sand so that the flow hits basically right in between the slits.
What’s going to happen with the sand?
Your intuition probably tells you that you will end up with two piles of sand of roughly the same size and shape, basically directly below the slits, right? That is what your intuition tells you, and also what should happen with light, if crazy old man Newton is to be believed. Grains of sand are particles, and they stand in for what Newton believed light to be- another, albeit much, much smaller, particle.
Thomas Young decided to test this, setting the stage for what has become one of the most famous experiments ever. Young proved that, far from behaving like a particle, light clearly showed interference patterns like a wave. This “so easy a caveman could do it” experiment was then double proven with the double slit experiment.
So this one’s also super easy, and you can do it at home. I know this, because I did it at home.
Similar to our sand example above, what you do is get a source of light (usually a laser pointer) and two slits close together on an opaque surface. You can actually buy a scientifically designed slide that is perfect for the experiment, or you can just cut 2 slits in aluminum foil with an exacto knife. (if you do that, make sure you’re using the non reflective side. And yes, aluminum foil has a .. well ok, a LESS reflective side.) Then aim the laser pointer at the slits.
Once again, what do you expect will happen? Do you think it will be like the sand thing, or something else? Well considering all this build up, I’m sure you know that the answer is “something else.”
You don’t get two bars of light illuminated on the wall like the sand. What you get is a long line of bright then dark then bright then dark bands on the wall called a interference pattern.
Like so:
(Note that there are a multitude of ways to generate interference patterns. The example above is done with a single thin thread of metal wire instead of two slits. This generates a pattern that is easier to photograph, but it is the same concept that is demonstrated by the two slit experiment.)
What's important to note is that the interference pattern that shows up on the wall is exactly what you would get if light was a wave. Waves cram themselves through the slits, and interfere with each other. A wave hits a wave, it makes a bigger wave (a bright spot). A wave hits a trough, it cancels out (a dark spot). Hence, the lighter areas and darker areas in the photo above, separated by a very even pattern. Furthermore, if you close one slit, the interference pattern vanishes, because there’s nothing for it to interfere with.
Note that if you want to reproduce this experiment, and see the wave interference pattern, you don't even need a laser point- go do the experiment on a calm body of water. take two floaty balls, and bounce them up and down in the water. Same thing happens- the waves radiate away from the balls, and interfere with each other in a very regular pattern.
So that settled that, right? Light is a wave? Well, yes, but- wait. I don't want to get ahead of myself. We need to talk about something extraordinary regarding light waves first.
PART 3: Light History: Photon Behavior Gets Complicated
So in order to create those interference patterns you need more than one wave right? I mean obviously. WELL … about that. We have always described light as a photon. When you point a laser at two slits, what you're doing is sending untold trillions of photons in that direction. Those photons hit one of the two slits, go through, interfere with each other, create patterns, etc etc etc. But if we fast forward our story just for a moment, we get to a point with experimental equipment where you can literally send only one photon at a time at the slits. What do you think you’re gonna get?
Well the answer is, unbelievably, you still get an interference pattern - it just takes a lot longer. to show up. If for example you put photosensitive paper behind the 2 slits, fire one photon at a time at the slits, and wait long enough, interference patterns will absolutely show up. How? How is this possible? What is a single photon going through the apparatus interfering with? To quote my main source for this article, the amazing book Through Two Doors at Once, “This is rather curious.” How’s that for an understatement?
We sent through a single photon, and it seems like it was interfering with itself. I’m not going to go too much further down this rabbit hole but I just wanted you to understand that the quantum realm is far, far weirder than has been imagined.
Furthermore, if you set up a mechanism wherein you would know for certain which slit the photon goes through, what do you think happens?
You guessed it. The interference pattern goes away. Light (or to be pedantic, photons) act as both a wave and a particle, and how it reacts depends on if it’s being observed or not. What's being observed in this case is the 'decision' (not a great word, I know) that the photon makes in terms of which slit to go through. There are far more complex experiments that prove this that are outside the scope of this article that prove this over and over- if we can observe the 'choice' (another not ideal word) the photon makes, it behaves as a particle. If we cannot observe, it behaves as a wave.
Quick aside: Sending one photon at a time through the apparatus does show something else important that bears attention (and is relevant for quantum computing): You can never know for sure where the photon will land. You can only calculate (and I promised there would be no math) you can only calculate the probability of it landing somewhere within the range established by the conditions of the slits and the photosensitive receiver. In a later article I will dig into this deeper as to how it relates to quantum computing, but the word of the day is probability.
PART 4: Light History: 1925, or, Is This Article Ever Going To End
Things get obtuse from here, and very very mathy. A lot of stuff happened understanding what atoms really looked like - spoiler alert… that image you have in your head of electrons is wrong. In 1924, Louis de Broglie made a shocking proposal: It wasn’t just light that behaved as both a wave and a particle: all forms of other matter did too. This was later proven to be true. In 1925, Matrix mechanics was developed by Heisenberg to formalize the mathematical descriptions of probabilistic calculations. Some of the most important and consequential physicists in the modern world made their names in this crazily productive period of time, including Schrodinger who invented wave mechanics to quasi replace matrix mechanics… In reality Matrix and wave mechanics are both still used. To quote someone far, far more well versed than myself in these matters, “The Matrix Mechanics was an algebraic approach employing the technique of manipulating matrices. The Wave Mechanics, in contrast, employed differential equations and had a basic partial differential wave equation at its heart.”
And there we have it- the short, short version of how we went from Newton to Quantum mechanics. Quantum mechanics, as proven to work over the past hundred years, shows clearly that all matter is simply composed of these small, indivisible … stuff that we call. Energy, mass, etc. It adds up to become atoms, which become molecules, which become Xboxes. Those quanta simply do not behave like the Xboxes. Quanta do things like superposition and entanglement, which make quantum computing possible. Xboxes just let you play fallout for 7 hours when you should be writing term papers.
PART 5: Appendix A
The amount of weirdness that I read and ultimately left out for clarity’s sake and a minimum of tangents was absolutely astounding. Like this: Did you know that there is a huge argument about the observer effect, to wit: the theory that if we don’t observe something, it doesn’t exist. Ergo, for certain arguments in quantum physics, the question “if a tree falls in a forest and no one’s there to hear it, does it make a sound” could theoretically have a different answer … if nobody could hear it then nobody could see it so… what tree? Einstein famously rejected this all through his life, with biographer A. Pais recalling “during one walk Einstein suddenly stopped, turned to me and asked whether I really believed that the moon exists only when I look at it.” which… I mean, fair question.